Routers and Photonics form Optical Internet
By: Sri Nathan, Qtera Corp.
A truly optical internet—free of bandwidth restrictions and spatial boundaries—is essential to network providers that want not only to manage the enormous boom in multi-service traffic, but also prosper from it. The optical Internet is built on a purely photonic transport backbone, replete with bandwidth management and facilities restoration capability. The service layer (Internet protocol [IP] layer) of the network links directly with the photonic transport layer (see Figure 1). This architecture packages and transports data traffic much more efficiently than networks do today, yielding significant benefits to carriers in terms of cost, scalability, restoration times, bandwidth provisioning, and overall footprint savings.
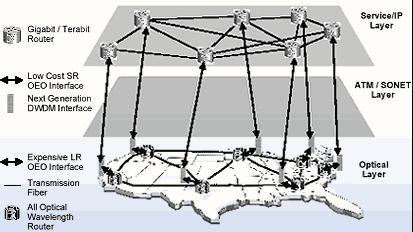
One critical difference between designing transport networks for IP traffic versus voice traffic is geography. Data has no respect for geographic boundaries. With a simple mouse click, Internet users trigger information flow between nodes that are thousands of miles apart. Conversely, voice networks generate revenue as a function of the distance over which traffic flows.
Carriers must forfeit the voice network's lucrative "distance surcharge" in a data-centric environment. Because of data traffic's distance independence, network providers with either established infrastructures or new data-centric networks need technologies that haul traffic over longer distances at a lower cost per bit than voice equipment. A photonic backbone supporting high-capacity routers accomplishes this better than a legacy network of synchronous optical network (SONET) equipment.
Spoiled legacy network
Today's view of a multi-tier network—service layer, SONET layer, and physical layer—is woefully outdated and will not be able to scale to meet the exploding volumes of future data traffic (see Figure 2). Because the data traffic pattern shows no (adjacent) community of interest, it is not conducive to popular SONET-layer architectures such as bi-directional line switched rings (BLSR). Any architecture that involves heavy SONET multiplexing incurs unnecessary optical to electrical conversion, resulting in higher cost.
In a multi-tier infrastructure, carriers often must include an "aggregation layer" of equipment to justify the costs of initiating one wavelength worth of traffic. Projected increases in traffic volume justify an express wavelength, with no aggregation, between many city pairs.
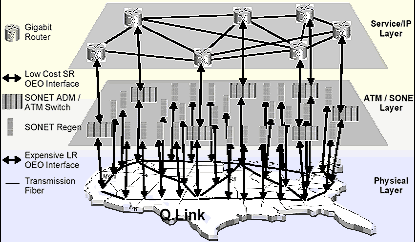
The idea of eliminating the SONET layer within the transport backbone has been germinating for some time. The strategy avoids electrical conversion of optical signals (i.e., SONET visibility) and minimizes the overall network cost. However, many of the solutions proposed to "eliminate" the SONET layer just replace that layer with another box. These interim solutions significantly reduce the cost benefits associated with a direct service-to-transport strategy, and insert unnecessary complexity into the backbone.
One interim solution to weaning networks away from SONET transport is to use huge wavelength cross-connects with electrical switch fabrics and high-speed (e.g., OC-48) interfaces and switching matrices. These cross connects are simply a consolidation of many SONET add/drop multiplexers (ADMs). Though wavelength cross-connects have a cost advantage over multiple SONET ADMs, the introduction of advanced IP router technology obviates the need for the devices. If routers perform the function of an Ultra Band DXC, an independent box adds cost and complexity to the overall network architecture.
A photonic transport system requires no opto-electronic (OEO) regeneration, thus eliminating the need for the most expensive part of today's long-haul backbone: Electrical regenerators and add/drop multiplexers (ADMs). Eliminating these network elements reduces capital costs and simplifies the network backbone. Additional savings arise from the elimination of back office systems, floor space, and other operating expenses needed to maintain this equipment. This helps reduce the overall costs of the transport network by as much as 40% compared with today's architectures (see figure 3).
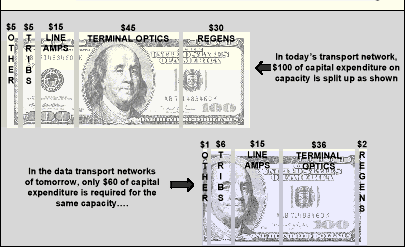
In order for this new two-tiered network to be truly viable, photonic networking systems must provide additional functionality, including ultra-long-reach transmission, facilities restoration, bandwidth provisioning, and performance monitoring. This new breed of photonic networking equipment is the second part of the puzzle that will comprise the optical Internet. In order to provide this additional functionality, photonic systems will take advantage of new advances in optical amplification technologies, new modulation schemes and high-performance ASICs designed specifically to push long-haul distances at terabit capacities.
The purely photonic backbone of an optical Internet does not exist yet. However, the advent of multi-gigabit and terabit routers (developed by such companies as Juniper, Avici, Cisco Systems Inc., and others), bring the second layer of an optical Internet closer to reality. These high-performance routers, which sit at the core of a provider's backbone network, take advantage of an all-optical networking environment.
When the service layer links directly to the transport layer, terabit routers will act as the integrating platform for multi-service network traffic, including leased line, voice, video, and data services. In other words, routers will be the first integral piece of the two-tiered network puzzle. High-performance routers feature high bit rate optical interfaces (OC-48c and OC-192c), which obviates the need for high bit rate multiplexing traditionally performed by a SONET terminal. Terabit routers also handle equipment protection, another SONET function, which further dilutes the need for SONET.
Two-tier benefits
Combining high-performance routers with advanced photonic networking equipment significantly improves scalability, restoration, and bit rate transparency.
Scalability is achieved in terms of a modular architecture, as well as flexible bandwidth. From an architectural design standpoint, direct IP-over-photonics implementation does not require non-blocking cross-connect matrices, which typically encounter port-count scalability restrictions. In terms of bandwidth, carriers must be able to provide very high bit rates to accommodate rapidly expanding data services. Transport systems that employ only 2.5 Gb/s DWDM channels are a dead-end solution. The baseline for next-generation photonic networks is 10 Gb/s, with 40 Gb/s on the near horizon.
The ability to offer tremendous amounts of bandwidth highlights another benefit of the routers + photonics model: Facilities restoration. When bandwidth was limited and expensive, optimization and efficient use of bandwidth were the primary objectives in cost-sensitive, long-haul networks. For this reason, complex restoration schemes were required to maximize the sharing of restoration-path bandwidth. Since this new, ultra long-reach architecture provides abundant bandwidth at a much cheaper cost per bit, long-haul carriers can use faster restoration methods, such as simple diverse routing at the optical layer. With this model, total failure detection and restoration times can be reduced to a few milliseconds.
Finally, a two-tier network architecture offers bit rate transparency. A purely photonic networking system will be able to interface directly to any bit rate supported by the router at the wavelength level, whether OC-12c, OC-48c, OC-192c or beyond.
The benefits of an optical Internet demonstrate the need for a simplified two-tiered network architecture—high-performance routers plus advanced photonic transport. While SONET will continue to perform critical functions in the access portion of the network, where distance and scalability are less immediate concerns, the transport network will no longer require an intermediate SONET layer. This long-haul transport network will evolve into something far more efficient and powerful—a purely photonic backbone for the Optical Internet.
About the author…
Dr. Sri Nathan is vice president of network architecture for Qtera, tel: 972-238-1740; E-mail: nathan@qtera.com.